Cashman Rocks Free Coins
Welcome to House of Fun's own bonus collector! Get your free coins, free spins, daily freebies and other giveaways right here on HoF!
Download any of these for free at DM me if you have any requests for anything not on the list. Shelly Cashman Series Microsoft Windows 10: Intermediate, 1st. Download any of these for free at DM me if you have any requests for anything not on the list. Shelly Cashman Series Microsoft Office 365 & Office 2016. 594.3k Followers, 50 Following, 1,774 Posts - See Instagram photos and videos from Jean-Luc Reichmann (@jean.luc.reichmann).
House of Fun Free Coins and Spins
Slot machines are one of the most popular forms of entertainment in the world yet playing them has traditionally posed a few obstacles. First, travelling to a casino typically takes some major planning and travel arrangements. Second, feeding coins into a slot machine at a traditional casino can really take a toll on your bank account if you're not careful.
Thankfully, House of Fun free spins are here to cut the inconvenience and stress out of your casino slots experience and inject a much-needed dose of excitement and gaming fun. Released every three hours, our free House of Fun coins ensure you always have a way to play your favorite slots games. Never be bored again with House of Fun freebies!
Free Coins Are Everywhere!
★ You can find Free Coins & Gifts in the game lobby, our Facebook page and our Instagram Feed.
★ Get the latest news and awesome Freebies with Notifications and emails.
★ And don’t forget to share the FUN with your friends by sending and receiving Coin Gifts.
HOF Free Coins = Free Spins
There’s nothing quite as satisfying as getting free stuff, especially when it involves a game that you love. When you redeem your House of Fun bonus coins, you can use these coins for free spins for your favorite slot games. Our hourly bonuses are your gateway to endless entertainment. Test your luck on one of our beautifully designed and immersive digital slot machines.
Feeling a bit bored? Whip out your favorite mobile device and use your free spins to pass the time in exhilarating fashion.
This is How House of Fun Freebies are Distributed
Are you wondering how to get free coins on House of Fun? Look no further! Keep up with all our House of Fun coins giveaway on Instagram, Facebook and through our daily emails that include House of Fun slots free coins. Earning free coins is as easy as following us on our social media channels, so you can always know when new HOF free spins are available.
Become a House of Fun bonus collector by signing up for our daily bonus emails, which always include free coins. Wake up to new ways to play House of Fun for free every day of the week. Trust us. You don’t want to miss a single one of our bonus offers.
House of Fun Bonus Daily Giveaway
Every three hours, House of Fun players can collect free bonus spins, just by loading the app. The more you play, the larger the prizes become. Play House of Fun free every single day and your free coins will grow by the hour, expanding exponentially until you receive the massive eighth day prize. After eight days of consecutive play, you start the process all over again, so you’ll always have access to free House of Fun coins.
In order to never miss a House of Fun giveaway, play our slots every single day and keep a close watch on our social media accounts. When it comes to earning free coins, consistency is key.
Be a Good Friend – Give Free Coins Gifts
Sharing is caring, which is why House of Fun allows you to send free coins to your friends. Get your friends started with free coins for House of Fun, or if they’re already House of Fun fans, keep them playing with even more free coins. House of Fun’s friend giveaway lets your buddies know you’re thinking about them, enriching your relationship and bringing you closer together.
Your friends will love playing House of Fun coins just as much as you do. Playing HOF slots is a great group activity, full of heart pumping action, edge of your seat excitement and intense joy. Play together and enjoy your unlimited coins House of Fun with your best bud.
Free Coins FAQ
How do you get free coins on House of Fun?
You can get Free Coins by collecting them every hour from the game lobby. Free Coins also come with notifications, emails, or gifts from your friends. To get even more freebies, follow House of Fun on Facebook and Instagram.
Does House of Fun pay real money?
No. House of Fun focuses on the pure thrill of exciting slot machines and rewarding challenges. Amazing virtual prizes, with real-life FUN.
Can you win real money in the House of Fun app?
No. There are no real money prizes in House of Fun, but there’s plenty to win and collect with daily challenges, exciting Albums, and much much more!
Can I win free coins online?
Yes! There are plenty of ways to win Free Coins in House of Fun. With daily Challenges, amazing Quests, and dozens of exciting slots, you can win Free Coins in the way that suits you most. Don’t forget about the Freebies in the game lobby, emails, notifications, and on the Facebook fan page.
Do I need to download anything to get free coins in an online casino?
No. Free Coins and Gifts are available in the game lobby, on Facebook & Instagram fan pages, and through emails and Gifts from your friends. If you use our mobile app you can get collect Freebies by checking HoF’s notifications as well!
Can your knowledge of volcanic rocks make you rich? Yes!! That is, if you combine it with knowledge of mineral deposits and global economic factors. Volcanoes directly or indirectly produce or host deposits of aluminum, diamonds, gold, nickel, lead, zinc, and copper. We use most of these materials everyday and, over the course of a lifetime, consume some of them (via the products we buy and use) in great amounts. This page provides a basic overview of the mineral deposits hosted in volcanic rocks.
Right: Photo shows alluvial diamonds that were eroded from the Argyle pipe. Photo courtesy of Grant Boxer.
Aluminum ore, called bauxite, is most commonly formed in deeply weathered rocks. In some locations, deeply weather volcanic rocks, usually basalt, form bauxite deposits. This sample of bauxite ore is from Western Australia.
Uses for Aluminum
Aluminum has a wide range of common uses. It is lightweight, strong (especially with alloys), and conducts heat well. Many kitchen items (pots and pans, foil, dishes) are made of aluminum. Most materials used for transportation use large amounts of aluminum: cars, trucks, boats, aircraft, and aircraft engines. Road signs and high-voltage power lines are also made mostly of aluminum.
World Supply of Aluminum
About 110 million tonnes of aluminum was produced in 1994. Australia produces most of the world's aluminum. Diagram from ITAM Bauxite by the Minerals Council of Australia.
Deposits of nickel sulfides are mined from greenstone belts in many ancient volcanic terranes. The ore is associated with ultramafic lava flows called komatiites. Komatiites have more than 18 weight % magnesium oxide (MgO) and large amounts of the mineral olivine. Komatiites are derived from melting in the mantle.
Komatiites have a unique texture called spinifex. The texture is an intergrowth of long, skeletal crystals of olivine and/or pyroxene. The texture is named for its resemblance to spinifex grass in Australia. Photo courtesy of Rob Hill, CSIRO.
Cross-section of a typical komatiite lava flow.
Simplified from Hill and others, 1989.
Cross-section of igneous lithologies and associated massive nickel sulfides in a komatiite lava flow.
Simplified from Hill and others, 1989.
Core in komatiite lava with adcumulate texture.
Photo courtesy of Rob Hill, CSIRO.
Uses for Nickel:
Nickel is commonly alloyed with other metals to provide resistance to corrosion and heat, and to add strength and hardness. These alloys are used for industrial and consumer goods. Most of the nickel produced is used to make stainless steel. Stainless steel contains about 8% nickel. Monel metal, a highly corrosion-resistant alloy, contains 67% nickel and is usedin ship building, food-processing equipment and in hospitals. Nickel is used by the military for armor plate. Nickel is used in alkaline batteries, dyes, insecticides and as a catalyst. Nickel is commonly used in coins.
World Supply of Nickel
The Sudbury District in Canada is the world's largest producer of nickel.
Another major nickel producer is in Western Australia and includes the Kambalda and MurrinMurrin deposits. MurrinMurrin consists of five deposits with a total of 11.9 million tonnes at 1.01% Ni and 0.07% Co.
In 1993, a large deposit of nickel-, copper-, and cobalt-sulfides (~150 million tons) was discovered at Voisey Bay on the northeast coast of Labrador, Canada. This deposit formed in magma chambers that fed large basaltic eruptions.
In 1994, about 875,000 tonnes of nickel was produced worldwide. Major producers are the Commonwealth of Independent States (former Soviet Union), Canada, New Caledonia, Indonesia, and Australia. Diagram from ITAM Nickel by the Minerals Council of Australia.
Photo shows gold jewelry recovered from the ash deposits of the 79 A.D. eruption of Vesuvius.
Gold forms in close association with volcanoes or is hosted in volcanic rocks. Three environments/styles are most common: gold in greenstone belts, gold in porphyry deposits, and gold in epithermal deposits.
Gold in Greenstone Belts
Gold is found in Archean (rocks older than 2.5 billion years) greenstone belts in Australia, southern Africa, and Canada. Greenstone belts are volcanic-sedimentary sequences, which include ultramafic rocks, dolerite, basalt, chert, sandstone, shale, tuff, banded iron-formation and other rock types. These rocks are very complex, having undergone metamorphism, folding, faulting, and shearing. Gold is most commonly found along the edges of greenstone belts and associated with structural features. Intensely altered and fractured basalt is a common host rock. The gold is though to be mobilized by hydrothermal solutions during regional metamorphism. The solutions probably contain only a few parts-per billion gold but great volumes of solution can precipitate their gold in a small zone with favorable chemical conditions. The deposit itself is usually a quartz vein that carries the gold or adjacent altered rock.
A classic example of gold hosted in greenstone is the Golden Mile in Kalgoorlie, Western Australia. The top diagram is a simple map that shows folds, faults, rock type, and mineralization. The bottom diagram is a cross-section of the deposit. Dolerite refers to dike rocks with plagioclase crystals in pyroxene crystals. By 1993, 40 million ounces of gold has been mined from the Golden Mile. Diagram from ITAM Gold by the Minerals Council of Australia.
Another example of gold hosted in greenstone is the St. Ives deposit near Kambalda, Western Australia. Three mines have produced more than 2.1 million ounces of gold from 1980-1993. Another 5.4 million ounces of gold remains in the deposits. The gold is found in altered rocks in all parts of the stratigraphic sequence. Diagram from ITAM Gold by the Minerals Council of Australia.
Gold in Porphyry Deposits
Gold and copper are found in ore bodies associated with porphry. Porphry is a general term applied to igneous rocks of any composition that contain conspicuous phenocrysts (crystals) in a fine-grained groundmass. The term is from a Greek word for purple dye and was first applied to a purple-red rock with phenocrysts of alkali feldspar that was quarried in Egypt. Diagram from ITAM Copper by the Minerals Council of Australia.
This type of deposit forms beneath stratovolcanoes and is associated with subduction zones. Erosion strips off overlying rocks to expose the mineralization. Gold and copper are found in sulfide minerals disseminated throughout the large volumes of intrusive rock (strictly speaking, this ore is associated with volcanic systems, usually not the volcanoes themselves). This requires large amounts of rock to be mined, often in open pits. The deposits are commonly 3-8 km across and copper may be less than 1% of the rock. Porphyry deposits are zoned in alteration (potassic ® sericitic ® argillic ® propylitic) and mineralization.
Gold in Epithermal Deposits
Epithermal refers to mineral deposits that form in association with hot waters. The deposits form within 1 km of the surface and water temperatures are about 50-200 degrees C. Shallow bodies of magma supply heat. The rising hot water carries dissolved gold and other elements. The water boils about 300 m below the surface and hydrogen sulfide gas escapes. This causes the gold to precipitate. The boiling zone is the target for mineral exploration. Veins commonly host the economic minerals. Diagram from ITAM Gold by the Minerals Council of Australia.
Lihir Island in Papua New Guinea
Lihir Island is an epithermal deposit discovered in 1982. The island is made of three volcanoes including Luise caldera, where the deposit formed. The rocks are trachybasalt lava flows, breccia, and tuffs.The mineralized rocks are highly altered. Most of the ore is in breccia thought to have been a boiling zone for rising fluids. The deposit formed between 350,000 and 100,000 years ago. It is estimated that the deposit contains 21.3 million ounces proven and probably another 42 million ounces as a geological resource. Most of the gold is fine particles in pyrite (FeS) grains. Hot springs and fumaroles are still active on the caldera floor.
Uses for Gold
Gold is rare, durable, chemically inert, and beautiful. These qualities make it useful for monetary exchange and investment, jewelry, and art.Gold’s high electrical conductivity, malleability, and ductility make it useful as an industrial metal. Gold is alloyed with silver, copper, nickel, palladium, zinc, and titanium to increase its tensile strength. Note: for gold, 1 carat = 1/24 part. Pure gold is 24 carat.
World Supply of Gold
Below is a pie diagram of major gold producers. In 1994, 2,296 tonnes of gold was produced. South Africa is the largest producer, followed by the USA, Australia, and the CIS (former Soviet Union). Diagram from ITAM Gold by the Minerals Council of Australia.
Diamonds are crystalline carbon and the hardest known substance.
This photo shows diamonds from the Argyle Mine in northern Western Australia.
Diamonds are brought to the surface from the mantle in a rare type of magma called kimberlite and erupted at a rare type of volcanic vent called a diatreme or pipe. Kimberlite is a gas-rich, potassic ultramafic igneous rock that contains the minerals olivine, phlogopite, diopside, serpentine, calcite, and minor amounts of apatite, magnetite, chromite, garnet, diamond, and other upper mantle minerals. Upper mantle xenoliths are found in some kimberlite and provide clues to the magma's origin. The source depth for kimberlite magmas is estimated at 200 km, more than twice as deep as the source region for most magmas. At a depth of 200 km the pressure is 60,000 times greater than the surface and the temperature is about 1500 C. Kimberlite magmas are rich in carbon dioxide and water which brings the magma quickly and violently to the surface. Most kimberlites occur as multiple intrusive events. Kimberlite was named for the rock associated with diamonds in Kimberley, South Africa.
Right: Simplified from Hawthorne (1975).
Kimberlite magmas form 'pipes' as they erupt. A tuff cone is at the surface and formed by base-surge deposits. In the subsurface, a funnel-shaped body narrows to a depth of hundreds of meters. The pipe (also called a diatreme) is filled with kimberlite, with or without diamonds (only 1 in 5 of the pipes at Kimberley contain diamonds).
Just how many diamonds are needed to make aa pipe economical? Some South African mines operate at 25 carats of diamond per 100 cubic meters of rock or about 2 grams of diamonds per 100 tons of rock. Because diamond has a specific gravity of 3.5 grams per cubic centimeter, 1 cubic centimeter of diamond weighs 16 carats. Picture a giant 100-ton ore truck full of kimberlite - that truck contains only half of a cubic centimeter of diamonds! Only about 35% of those diamonds are gem quality.
Macles diamonds from the Argyle pipe, Western Australia.
Photo courtesy Grand Boxer.
Uses for Diamonds
Most diamonds are used in drill bits and diamond tools. A small number are used for glass cutters and surgical instruments. Only the finest are used as gems.
World Supply of Diamonds
Australia is currently the world's largest producer of diamonds. Most of these diamonds are low quality and used for industrial purposes. Most of the diamonds are from the Argyle diamond pipe in northern Western Australia. The pipe at Argyle is made of lamproite, not kimberlite. The mine produced 27.8 million carats (1 ct = 200 mg; 5 carats = 1 gram) of low grade diamonds in 1993-1994. Because of the high rate of production at Argyle, mining operations will end within the next few years. The mines at Kimberley, South Africa have produced a total of more than 200 million carats since the 1870s. About half of South Africa’s diamonds are gem quality.
Zinc-Copper volcanogenic massive sulfide (VMS) deposits have been observed as they form at mid-ocean ridges. Chimneys formed at the ridges have as much as 29 weight % zinc and 6 weight % copper in sulfide minerals (pyrrhotite, pyrite, sphalerite, and chalcopyrite). The minerals are dissolved in fluids at temperatures as high as 380 C flowing at 1-5 m/sec.
The minerals precipitate as the hot solution comes in contact with cold sea water. Photography courtesy of Woods Hole Oceanographic Institution and members of the Adventure dive (Principle Investigators: D. Fornari, R. Haymon, K. Von Damm, M. Perfit, M. Lilley, and R. Lutz).
Lead, zinc, and cooper are found in VMS deposits. The deposits form in deep ocean water by the precipitation of sulfide minerals released by submarine volcanoes.
Volcanic fluids and hot seawater move through the volcanic rocks and leach metals. The deposits are associated with lava flows, breccia, water-deposited tuffs, cherts, sulfates, and limestones. VMS deposits are usually associated with quartz, anhydrite, gypsum, and barite. This photo shows pyrite in silicified tuff from a VMS deposit in eastern Java, Indonesia. The sample contains 0.55 ppm gold.
There are three types of VMS deposits:
Zinc-Copper | Lead-Zinc-Copper | Copper-Pyrite | ||
Host rock | basalt to rhyolite | rhyolite | basalt or ultramaficlava | |
Age | Archean | Proterozoic-Paleozoic | Mesozoic | |
Ore minerals | sp, ch, py | ga, sp, py, some ch | ch, py | |
Associated metals | gold, silver | silver, some gold | gold | |
Famous Occurrences | greenstones of Canada, Australia | Sudbury and Bathurst Canada | Kuroko, Japan | Cyprus |
Setting | spreading centers | back-arc basins | spreading centers | |
Minerals: | sp = sphalerite | ch = chalcopyrite | py = pyrite | and ga = galena. |
Kuroko-style VMS deposits are found in dacite-rhyolite domes that erupted in the deep water of back-arc (behind the main volcanic arc) basins. Kuroko deposits are zoned from copper-rich near the center, to zinc-rich, to lead-rich at the outer edges of the deposit. The fluids that form Kuroko deposits have twice the salinity of average ocean water and temperatures of 250-300 C. This cross-section of a typical Kuroko deposit is from Sato (1974) and Franklin and others (1981). Modern-day VMS deposits of the Kuroko-style have been observed as they form in the back-arc basin of the Okinawa Trough (Halbach and others, 1989).
The deposit at Bathurst in Canada is an example of lead-zinc-copper VMS. Hydrothermal solutions associated with Keweenawan flood basalts have produced copper deposits in the Lake Superior area. Between 1845 and 1968, over 13 billion pounds of copper and 16 million ounces of silver were produced from the Keweenawan district.
The porphry (not VMS) mine at El Abra in Chile will produce 500 million pounds of copper annually.
Uses for Copper, Lead, and Zinc
Copper was used as long ago as 8000 BC for tools, weapons, and ornaments. The discovery of bronze (copper and tin alloy) about 3500 BC marked the onset of the Bronze Age. Romans used lead for the plumbing and sewage systems. In Modern times, the electrical conductivity, ductility, and resistance to corrosion of copper, lead, and zinc, make them very useful in alloys. Copper is used for electrical applications (50%), general and industrial engineering applications (20%), building and construction (15%), transportation (11%) and other applications. Lead is used in storage batteries, paints, dyes, explosives, insecticides, and rubber products.
Zinc is used in galvanized steel, protective coatings for steel, and die casting. Zinc compounds are used for luminous dials, cosmetics, plastics, rubber products, soaps, and inks.
World Supply of Copper
In 1991, 9,167,000 tons of copper was produced. Chile (19.8%), USA (17.8 %), and the CIS (9.8%) are the main producers. USA, Japan, and the CIS are the main consumers.
OBSIDIAN IS HOT STUFF
By Jim Miller, B.Sc., M.Sc. Geology*
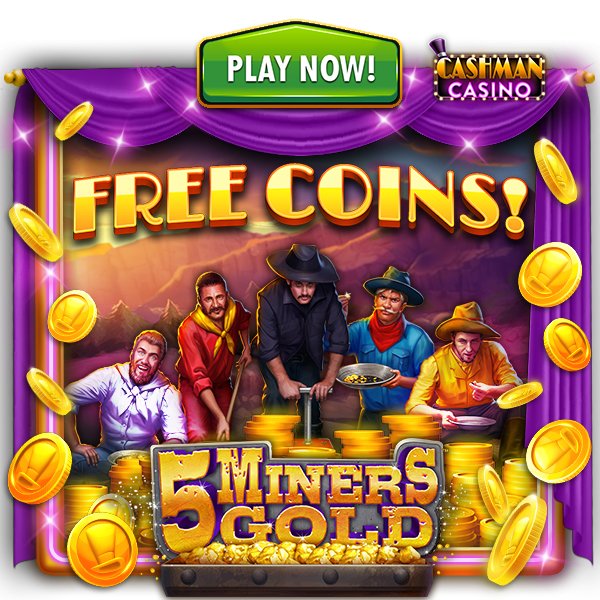

* Jim Miller is a practicing geologist and an avid flint knapper residing in Bothell, Washington. Examples of obsidian from Glass Buttes and stone tools made from those materials by the author are included with this article. |
|
(By K. Weldon, 2010)
Formation of That Black Glass Obsidian
Volcanic glasses such as obsidian form when some physical property of lava restricts ion mobility preventing an ordered crystalline pattern to develop, and for obsidian it is the viscosity that has the greatest control on the ordered crystalline pattern, the measure of viscosity is dependent on the temperature, crystal content and chemical composition . Viscosity is a measure on the ability of substance to flow, high viscosity means poor ability to flow and low viscosity means good ability to flow, an example of magma with a low viscosity is basalt and magma with high viscosity is rhyolite.
For obsidian to form, magma is trapped below the eutectic, point of crystallization, by loss of heat. Therefore leaving a magma that is unable to crystallize will form (glass) obsidian. For this process to occur during a lava flow the lava is caught just below crystallization temperature, thus forming a glass due to the inability to form a crystalline solid. The formation of obsidian could also be the melt, liquid remaining from a magma after crystallization, of a rhyolite magma that has been erupted before any crystals can form as stated earlier. The gas content of obsidian is very low so for this to occur the gas has to be released in some way before the eruption of the obsidian.

Obsidian occurs as a flow, not as an explosive eruption in contrast to a vesiculated rhoylite pumice or dacite. This difference is due to the difference in composition, specifically volatile, gas, content. Volatiles within highly viscous magmas can produce eruptive events due to the inability for the volatiles to escape easily so as they rupture, burst, they release an enormous amount of pressure producing an eruption such as the Mount. St. Helens eruption on May 18th 1980. For these explosive types of eruptions not occur in the emplacement of obsidian the volatile content for the obsidian must be low. The average water content of obsidian is (0.3 wt %) where as crystalline rhyolite is <2.0 wt % water (Bakken Barbara., 1977). The low amount of water in comparison with rhyolite pumice indicates that the flow of obsidian must take place at the end stage of the explosive eruption phase of rhyolite magma (Bakken Barbara., 1977) end stage indicating after the vent of the volcano has released a large amount of gas through the explosive eruption stage. When it comes down to it the temperature ot the obsidian magma is the may property controling the viscosity over any of the other properties.
The viscosity of obsidian must be lower than rhyolite so it can flow; difference in eruption temperature is the greatest control over this difference. Initially the magma erupts at a temperature around 900 degrees C however this first eruption is rich in volatiles producing pumice, so this stage still has a greater viscosity than obsidian. As volatile as pumice is released and the obsidian melt from the rhyolite is built up at a temperature around 900-700 degrees C is than released as the obsidian at a low viscosity of a magnitude of 10^8 Pa s, which is an order of 3 magnitudes less than that of the original rhyolite pumice eruption, this therefore indicates that the eruption of the obsidian has a similar temperature of eruption as the initial pumice only difference is in the magma's gas content. After obsidian has been erupted what to follow is typically a rhyolite dome rich in crystals due to slow cooling of the magma; this event makes it clear that the reason for lack of crystals in the obsidian are due to being erupted at a temperature greater than the final rhyolite magma. This information comes to the conclusion that the flow of obsidian is dependent on the time for the obsidian magma melt to cool, and that amount of time for cooling is very short because if allowed to cool to a temperature lower than initial temp it will not flow.
Bibliography:
- Bakken, B., (1977), Obsidian and Its Formation. North West Geology. 6-2, (88-92)
A model for Obsidian Flow emplacement
As a magma body of rhyolite composition approaches the surface volatiles exsolve, separate, from the magma and rise to the plumes top. The decrease in the confining pressure leads to frothing of the volatile rich top section of the plume as ascension of the stratified mass occurs. This process of vesiculation leads to some number of explosive eruptions depositing tephra. After these eruptions the remaining volatile rich magma reaches the surface as highly inflated pumiceous lava. The transition from the explosive eruption to the pumiceous lava occurs once gas pressure in vesicles can no longer exceed strength of magma.
Later in the eruption following the pumiceous lava flow the event of the emplacement of obsidian occurs, this process happens with the bubble free obsidian flowing out over the earlier emplaced coarsely vesicular pumice (highly inflated pumiceous lava). The Obsidian is slightly less viscous but more dense than the coarse pumice. There remains a gradual transition from the coarse pumice to obisidian due to volatile decrease with the progression from pumice phase to obsidian phase. Essentially while obsidian is erupted vesicular material continues to be extruded.
As the obsidian rides over the coarse pumice, volatiles continue to evolve from the cooling upper flow surface of the obsidian flow. This process of the volatiles exsolving from the obsidian flow forms a finely vesicular layer that keeps the flow interior of the obsidian insulated and warm; this contact is also gradational, just as the coarse pumice to the obsidian was.
Within the volcanic vent that the obsidian flowed from the remaining magma from within cools and crystallizes and then erupts as a crystal rich rhyolite flow capping the vent mouth as a rhyolite dome.
The Big Obsidian Flow of Newberry Volcano, Central Oregon is very similar in the process of emplacement. Firstly the eruption began with explosive eruptions depositing pumice and lava blacks of 1 meter (3 feet) in diameter, after these eruptions pyroclastic flow occurred essentially taking the place of the pumiceous lava flow, however there remain deposits of course pumice within the region of Big Obsidian Flow which many indicate a short event of pumiceous flow. The final stage was the emplacement of Big Obsidian Flow that moved very slowly and according to Sherrod et al (1997) this lava flow probably moved a few meters to tens of meters a day. From observations in the field of the Big Obsidian Flow the presence of a thin visculated layer coats some of the surface of the obsidian flow. The final stage of the emplacement of a rhyolite dome is not present the vent is actually plugged by the obsidian flow indicating that the rhyolite stage was not present in the eruption or possibly the rhyolite magma was already to cool and harden to come to the surface of the flow.
This is an image of the Big Obsidian Flow of Newberry Volcano,a USGS Photograph taken in October 1987 by Willie Scott. The structure of the deposit indicates that the obsidian flowed over being erupted explosively.
Fink (1983) provided most of the information regarding the flow emplacement model of obsidian as well as the figures displayed.
Obsidian occurs as a flow, not as an explosive eruption in contrast to a vesiculated rhoylite pumice or dacite. This difference is due to the difference in composition, specifically gas content. Gases within highly viscous magmas can produce eruptive events due to the inability for the volatiles to escape easily from the magma so as they rupture they release an enormous amount of pressure producing an eruption such as the Mount. St. Helens eruption on May 18th 1980. For these explosive types of eruptions not occur in the emplacement of obsidian the gas content of the obsidian must be low. The average water content of obsidian is (0.3 wt %) where as crystalline rhyolite is <2.0 wt % water (Bakken Barbara., 1977). The low amount of water in comparison with rhyolite pumice indicates that the flow of obsidian must take place at the end stage of the explosive eruption phase of rhyolite magma (Bakken Barbara., 1977) end stage indicating after the vent of the volcano has released a large amount of gas through the explosive eruption stage. When it comes down to it the temperature ot the obsidian magma is the property controling the viscosity over any of the other properties, higher the temperature the lower the viscosity.
The viscosity of obsidian must be lower than rhyolite so it can flow; difference in eruption temperature is the greatest control over this difference. Initially the magma erupts at a temperature around 900 degrees C however this first eruption is rich in volatiles producing pumice, so this stage still has a greater viscosity than obsidian. As volatile rich pumice is released and the obsidian melt from the rhyolite is built up at a temperature around 900-700 degrees C the obsidian melt can be erupted at a low viscosity of a magnitude of 10^8 Pa s, which is an order of 3 magnitudes less than that of the original rhyolite pumice eruption, this therefore indicates that the eruption of the obsidian has a similar temperature of eruption as the initial pumice only difference is in the magma's gas content. After obsidian has been erupted what to follow is typically a rhyolite dome rich in crystals due to slow cooling of the magma; this event makes it clear that the reason for lack of crystals in the obsidian are due to being erupted at a temperature greater than the final rhyolite magma. This information comes to the conclusion that the flow of obsidian is dependent on the time the obsidian is erupted during the eruption, the temperature of the obsidian and the gas content.
Bibliography:
- Fink, J.H., (1983), Structure and emplacement of a Rhyolite Obsidian Flow: Little Glass Mountain, Medicine Lake Highland, Northern California. Geological Society of America Bulletin. 94 (362-380)
- Sherrod., Mastin., Scott., Schilling. (1997). Newberry caldera, Oregon Big Obsidian Flow - Latest Eruptive Event Excerpt from: Sherrod, Mastin, Scott, and Schilling, 1997, Volcano Hazards at Newberry Volcano, Oregon. U.S. Geological Survey Open-File Report, 97(513)
Flow Textures and Features
Obsidian flows usually consist of fold surfaces, explosion craters, flow banding, and cavities underling the craters.
The explosion craters are commonly located on the surface of the flow and can have diameters of 10-25m (36-91ft), at 5-15m (15-55ft) deep; the accompanying cavities can be 5-15m in length. The cavities form near the surface by a buckling mechanism. Buckling occurs by contrast of stiff upper layer relative to the near liquid flow interior, which forces the upper layer to make an almost triangular shape at the surface, separating it from the lower layer. These cavities can collect magmatic/meteoric water*, and as vapor pressure builds up and exceeds the strength of the surface crust of cavity an explosion results producing the explosion caters.
*Meteoric water: rain water/ground water, not from the magma.

Image depics the event of cavities forming by buckling and exploding due to gas pressure exceeding the strength of the rock. This illustration comes from Castro's Structural origin of large gas cavities in the Big Obsidian Flow, Newberry Volcano.
Flow banding in obsidian is a distinctive characteristic and the abundance of the flow banding. Even with the abundance of these flow bands within obsidian little is known about the origins of these features, but what is known is that these freatures derive from both crystallization and deformation processes. According to a paper by J.M. Castro the flow bands develope from the deformation of microlites (very small phenocryst) by shear strains during the flow of the obsidian. The picture below shows the flow banding as pointed out by the arrow.
Bibliography
Hydration of Obsidian
After an obsidian flow has been emplaced it is subject to the atmosphere that causes weathering to the obsidian. One specific type of weathering done on to obsidian is called hydration, which occurs by the water within the atmosphere being absorbed by the obsidian thus increasing the water content within the rock. The product of a hydrated obsidian is called devitrified obsidian, (de- to remove) (vitrify- glassy), so essentially the removal of the glassy property of the obsidian. The products of devitrified obsidian can produce secondary fiberous mineral crystals that can form ball like shapes called spherulites that are embedded within the obsidian, these secondary minerlas can develope into what is called snowflake obsidian as well, which makes the obsidian look as if it is decorated with snowflackes due to the snowflake shapes the secondary minerals produce. The snowflake obsidian can be used as jewlery and many rockhounds search for it, Yellowstone caldera is home to snowflake obsidian.
This is an image of snowflake obsidian, as you can see the seconday minerals group together forming snowflake like shapes scattered across the rock surface. This image is from Rockhoundblog.com.
This is an image of what a spherulite looks like due to secondary mineralization from devitrification of obsidian.
Photo by (c) 2008 Andrew Alden, licensed to About.com
New Research concerning Hydration of Obsidian
Some researchers have used the hydration rate of obsidian to acquire specific dates of the obsidian, which is based on the idea that a freshly broken obsidian surface begins to absorb water from its environment almost immediately. Absorption continues with time, generating a hydrated layer whose thickness is proportional to the time the glass surface was exposed this according to Anovitz et al (2006). This technique of dating can be unreliable due to the many factors influencing rate of hydration. Just recently Anovitz et al discovered that the natural hydration of obsidian can be used as a tool to provide paleoclimatic reconstructions. Anovitz et al (2006) article in GSA’s Geology Journal, “Obsidian hydration: A new paleothermometer,” the first successful application of this idea was done on samples from the the Chalco site in the Basin of Mexico successfully obtaining the temperature change within the region of study, for further information refer to the Anovitz (2006) article.
Bibliography:
Anovitz, L. (2006). Obsidian hydration; a new paleothermometer. Geology [Boulder], 34(7), 517-520. doi:10.1130/G22326.1.
Francis, Peter., and Oppenheimer, Clive. (2004), Volcanoes, Second Edition. New York: Oxford University Press Inc., New York: (162-164).
Ophiolites are pieces of oceanic plate that have been thrusted (obducted) onto the edge of continental plates.
They provide models for processes at mid-ocean ridges.
Ophiolites are an assemblage of mafic and ultramafic lavas and hypabyssal rocks found in association with sedimentary rocks like greywackes and cherts. They are found in areas that have complex structure. Cross-sections simplified from R.C. Coleman, 1981, Journal of Geophysical Research, v. 86, p. 2497-2508.
Ophiolites have been found in Cyprus, New Guinea, Newfoundland, California, and Oman. The Samail ophiolite in southeastern Oman has probably been studied in the greatest detail. The rocks probably formed in the Cretaceous not far from the what is now the Persian Gulf. The rocks were later thrust (pushed uphill at a low angle) westward onto the Arabian shield.
Ophiolites are characterized by a classic sequence of rocks. This sequence is well exposed at the Samail ophiolite. The base of the sequence is sedimentary rocks of the Arabian shield, not part of the ophiolite, on which the oceanic plate was pushed. From base to top the ophiolite is made of: peridotite, layered gabbro, massive gabbro, dikes, and volcanic rocks. At Samail this entire sequence is 15 km thick. The basal peridotite is made of a rock called harzburgite (made mostly of the minerals olivine and enstatite). Within the peridotite are many dikes of gabbro and dunite. The peridotite is deformed. The peridotite is overlain by dunite (an intrusive igneous rock made mostly of the mineral olivine) that grades upward to gabbro (an intrusive igneous rock made mostly of plagioclase and clinopyroxene - augite). The sequence is capped by dikes and volcanic rocks (pillow basalts that erupted on the ocean floor). Sequence of rocks simplified from R.C. Coleman (1981).
From a tectonic perspective, the peridotite is depleted mantle that was under the magma chamber at the mid-ocean ridge crest. The gabbro layer is related, in some way, to the crystallization of the magma chamber (probably with repeated injections of magma). The dikes and volcanic rocks are formed by magma in transit to or at the surface. Cross-section simplified from Pallister, J.S., and Hopson, C.A., 1981, Journal of Geophysical Research, v. 86, p. 2593-2644.
To see a classic bit of oceanic crust that has been thrusted up on a continent (an ophiolite ) visit the Oman Virtual Field Trip.
Relevant peer reviewed journal sources include:
Barker, D.S., 1983, Igneous Rocks: Prentice-Hall, New Jersey.
Boxer, G.L., and Jaques, A.L., 1990, Argyle (AK1) diamond deposit, in Geology and Mineral Deposits of Australia and Papua New Guinea, Hughes, F.E., ed., The Australian Institute of Mining and Metallurgy, Melbourne, p. 697-706.
Boyle, R.W., 1979, The geochemistry of gold and its deposits: Geological Survey of canada Bulletin 280, 584 p.
Cox, K.G., 1978, Kimberlite pipes: Scientific American.
Evans, A.M., Ore Geology and Industrial Minerals: Blackwell Science, 389 p.
Franklin, J.M., Lydon, J.W., and Sangster, D.F., 1981, Volcanic associated massive sulphide deposits: Economic Geology 75th Anniversary Vol., p. 485-627.
Guilbert, J.M., Park, C.F., Jr., The geology of Ore eposits: W.H. Freeman and Company, 985 p.
Cashman Rocks Free Coins Price Guide
Halbach and others, 1989, Probable modern analogue of Kuroko-type massive sulphide deposits in the Okinawa Trough back-arc basin: Nature, v. 338, p. 496-499.
Hawthorne, J.B., 1975, Model of a kimberlite pipe: Phys. Chem. Earth, v. 9, p. 1-15.
Hill, R.E.T., Barnes, S.J., Gole, M.J., and Dowling, S.E., 1987, Physical volcanology of komatiites: Excursion Guidebook No. 1: Geological Society of Australia, 99 p.
Hill, R.E.T., Barnes, S.J., and Perring, C.S., 1996, Komatiite volcanology and volcanogenic setting of associated magmatic nickel deposits, in Nickel ’96, Australian Institute of Mining and metallurgy Publication Series No 6/96, p. 91- 95.
Hill, R.E.T., Gale, M.J., and Barnes, S.J., 1989, Olivine adcumulates in the Norseman-Wiluna greenstone belt, Western Australia: Implications for the volcanology of komatiites: in Magmatic Sulphides - the Zimbabwe volume (ed., M.D. Prendergast): The Institution of Mining and metallurgy: London, p. 189-206.
Hill, R.E.T., Barnes, S.J., Gole, M.J., and Dowling, S.E., 1987, Physical volcanology of komatiites: Excursion Guidebook No. 1: Geological Society of Australia, 99 p.
Jaques, A.L., Lewis, J.D., and Smith, C.B., 1986, The kimberlites and lamproites of Western Australia: Geological Survey of Western Australia Bulletin 132, 268 p.
Meeker, K. A., Chuan, R. L., Kyle, P. R., and Palais, J. M., 1991, Emission of elemental gold particles from Mount Erebus, Ross Island, Antarctica. Geophys. Res. Lett., 18, 1405-1408.
Ohmoto, H., 1996, Formation of volcanogenic massive sulfide deposits: The Kuroko perspective: Ore Geology Reviews, v. 10, p. 135-177.
Sato, T., 1974, Distribution and geologic setting of the Kuroko deposits: Society. Min. Geol. Japan Special Issue 6, p. 1-9.
Sawkins, F.J., Metal Deposits in Relation to Plate Tectonics: Springer Verlag, 461 p.
Sillitoe, R.H., 1973, The tops and bottoms of porphyry copper deposits: Economic geology, v. 68, p. 799-815.
Tatsumi, T., 1970, Volcanism and Ore genesis: University of Tokyo Press.
Whitney, J.A., and Naldrett, A.J., Ore Deposition Associated with Magma: Reviews in Economic Geology, v. 4. 250 p.
Other excellent sources of information include:
If you need a summary of the nature of any element visit the Webelements.
If the mineral names are confusing visit Minerals by Name to learn more about each mineral.
United States Geological Survey Mineral Resources describes mineral resources in different regions.
Natural Resources Canada has numerous interesting pages including Minerals and Metals at Home!
The Minerals Council site provides current general information and educational material about the Australian minerals industry.
The Nickel Institute provides information on the uses and properties of nickel.
The Copper Page provides information on the uses and properties of copper.
Timothy J. Barrett and Ore Systems Consulting describe several VMS deposits in British Columbia.
1. On a daily basis, most of us use the following metals mined, at least in part, from volcanic ore deposits:
_Aluminum
_Zinc
_Copper and Lead
_Nickel
_All of the Above
2. Which of these economic materials are found in kimberlite pipes:
_Aluminum
Cashman Rocks Free Coins Slot Machine
_Gold
Get Free Us Coins
_Copper, Lead, Zinc
_Diamonds
_Nickel
3. Which of these economic materials are formed by hydrothermal fluids at submarine volcanoes:
_Aluminum
_Gold
_Copper, Lead, Zinc
_Diamonds
_Nickel
4. Which of these economic materials can be produced by deep weathering of basalt:
_Aluminum
_Gold
_Copper, Lead, Zinc
_Diamonds
_Nickel
5. Which of these economic materials are found in komatiite lava flows:
_Aluminum
_Gold
_Copper, Lead, Zinc
_Diamonds
_Nickel
Free Cashman Coins Facebook
6. Porphyry deposits are found in:
_Extensive Lava Tube Systems
_The Eroded Plumbing Systems of Volcanoes
_Ophiolites
_Kimberlite Pipes
_Grand Forks, North Dakota (home of the fighting Sioux!)
7. Diamonds form under the following conditions:
_pressure and temperatures similar to the Earth's surface
_pressure 60 times greater than the surface and a temperature of about 150 C
_pressure 60,000 times greater than the surface and a temperature of about 1500 C
_pressure and temperatures similar to the Earth's core
8. As a nest-egg for your retirement would it be best to have a kilogram of:
_Aluminum
Cashman Casino Coins Free
_Gold
_Copper
_Diamonds
1. On a daily basis, most of us use the following metals mined, at least in part, from volcanic ore deposits:
e. all of the above
2. Which of these economic materials are found in kimberlite pipes:
d. diamonds
3. Which of these economic materials are formed by hydrothermal fluids at submarine volcanoes:
c. copper, lead, zinc
4. Which of these economic materials can be produced by deep weathering of basalt:
a. aluminum
5. Which of these economic materials are found in komatiite lava flows:
e. nickel
6. Porphyry deposits are found in:
b. the eroded plumbing systems of volcanoes
7. Diamonds form under the following conditions:
c. pressure 60,000 times greater than the surface and a temperature of about 1500 C
8. As a nest-egg for your retirement would it be best to have a kilogram of:
d. diamonds